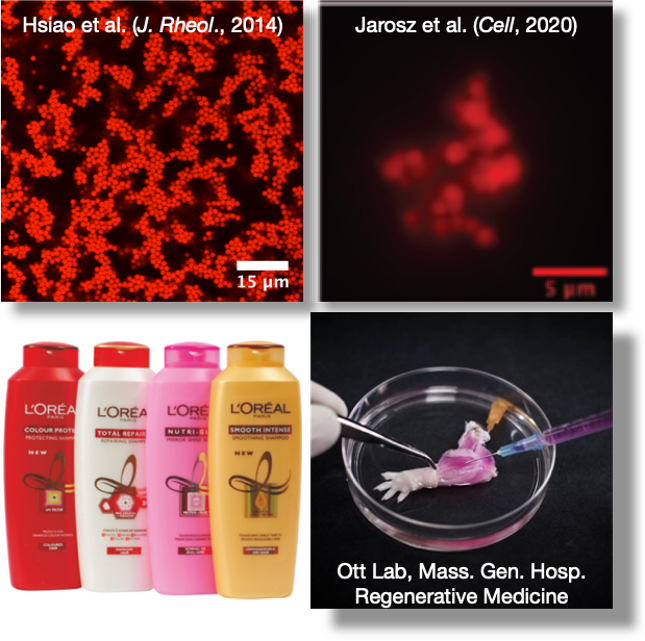
Colloidal gels are ubiquitous because their multiphase structure – millions of micron-sized particles interconnected by physical bonds and suspended in a liquid – imparts reversible liquid-like and solid-like behavior. Colloidal gels form when phase separation fails, freezing the condensing region into a bonded network of strands via a kinetic process thermodynamic theory cannot predict.
The consensus view labeled colloidal gels as static, arrested materials that rupture like solids. However, this resulted in long-standing shortfalls in the ability to predict and engineer their behavior. My group developed a model of gel “Phase Mechanics” that explains these behaviors.
“Phase Mechanics” of colloidal gels
Our group constructed the first large-scale computational model of a reversible colloidal gel, along with a suite of micromechanical algorithms to age and interrogate the gel down to the particle scale. From this we proved that reversible gels, which are placed on equilibrium phase diagrams and identified as being in “arrested phase separation,” instead continue to evolve; that age coarsening pushes toward more complete phase separation but simultaneously deepens arrest; and that gel yield is actually a release from so-called arrest and a leap forward in phase separation.
We determined the long-sought mechanistic origin of gel collapse: a reactivation of negative osmotic pressure that releases gels from their apparent arrest and furthers their phase separation. We showed that yield can occur by rupturing fewer than 0.1% of network bonds, which permits many other bonds to relax, triggering a cascade of relaxation. We synthesized our findings into “Phase Mechanics,” a framework for gel birth, aging, death and re-birth that casts each stage as a kinetic path through the phase diagram. Related publications: Zia, Landrum & Russel, J. Rheol. 2014; Landrum, Russel & Zia, J. Rheol 2016; Padmanabhan & Zia, Soft Matter 2018; Johnson, Landrum & Zia Soft Matter 2018; Johnson, Moghimi, Petekidis & Zia, J. Rheol. 2019.’
Vitrification in colloidal glasses
Despite decades of study, the mechanistic process of colloidal vitrification has remained murky. Prevailing theories and experimental reports suffer two fatal flaws: divergence is prescribed to occur and the cooperative motion claimed as the mechanism of relaxation requires large-scale structure that does not exist. Both issues emerge from the difficulty of quenching deep into the glass. My group overcame this barrier by developing a novel size-jump algorithm. With this we showed that dynamics persist up to maximum packing, disproving the notion of divergence or a thermodynamic glass transition.
By examining a broad range of wave numbers and monitoring physical displacement, my group’s work established a new mechanism for glassy relaxation: Correlated motion relaxes the glassy plateau and enables long-time, short-range “dense” self-diffusion that relaxes and ages the glass to the intransient state. Related publications: Wang, Li, Peng, McKenna & Zia, Soft Matter 2020; Peng, Wang, Li, Chen, Zia & McKenna, Phys. Rev. E 2018.
Damascus colloids
Building and expanding on our expertise in micromechanical models, we aim to create completely new soft solids by intervening in phase “arrest” using quenching and annealing techniques. In collaboration with the Helgeson Lab (UCSB), we are embarking on a combined experimental and theoretical examination of colloidal microstructure evolution during complex kinetic trajectories through regions of phase instability en route to kinetic arrest. Leveraging these ideas to design colloidal soft matter will enable a dramatic expansion of configurable materials for artificial tissue scaffolds, advanced membranes, and shape memory materials.